Hydroponics a Standard Methodology for Plant Biological Researches
Hydroponics is a critical methodology in plant biological research due to its enhanced control over nutrient delivery and environmental conditions. This soilless cultivation method reduces water usage by up to 90% and accelerates growth rates by up to 50%, compared to soil-based systems.
Hydroponics allows precise monitoring of pH, electrical conductivity, and nutrient composition, ensuring ideal plant health and reliable experimental outcomes. It is indispensable for genetic, stress response, and biochemical studies, offering high data consistency and reproducibility.
Understanding hydroponics’ scientific framework will illuminate its pivotal role in advancing plant biology research.
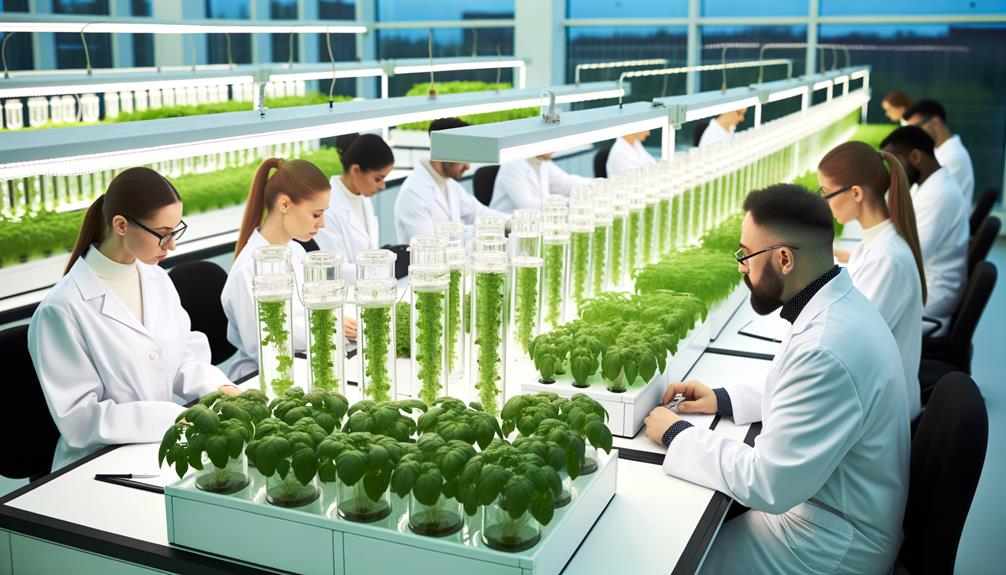
Key Takeaways
- Hydroponics offers precise control over nutrient delivery, optimizing plant health and growth.
- Water consumption in hydroponics is up to 90% less than traditional soil methods.
- Hydroponic systems minimize soil-borne pathogens and pests, ensuring cleaner research conditions.
- Controlled environments in hydroponics enhance reproducibility and accuracy in genetic and stress response studies.
Key Aspects of Hydroponics in Plant Biological Research
Aspect | Description | Research Applications |
---|---|---|
Definition | Hydroponics is a method of growing plants without soil, using mineral nutrient solutions in water. | Commonly used in plant physiology and genetics studies to control variables such as nutrients and water. |
Nutrient Control | Precise control over the nutrient mix provided to plants. | Enables researchers to study nutrient uptake and its effects on plant growth and development. |
Water Usage | Efficient water use, often using less than traditional soil-based methods. | Useful for drought studies and research into water-efficient crop systems. |
Growth Rate | Faster plant growth due to optimized nutrient and water conditions. | Studies focusing on plant productivity and the effects of environmental stressors. |
Environmental Control | Allows researchers to create controlled environments to study the effect of different variables. | Ideal for studies on climate change impacts, disease resistance, and adaptation to changing conditions. |
Automation Potential | Can be highly automated, reducing labor and increasing data precision. | Supports high-throughput research and long-term studies on plant growth cycles and productivity. |
Common Plants Studied | Leafy greens, herbs, tomatoes, and root vegetables. | Used to study a wide variety of crops, from basic biology to large-scale agricultural applications. |
Benefits of Hydroponics

Hydroponics offers a myriad of advantages, including enhanced control over nutrient delivery, accelerated plant growth rates, and significant reductions in water usage compared to traditional soil-based cultivation methods.
This cultivation technique allows precise manipulation of macro and micronutrient concentrations, facilitating ideal plant health and productivity.
Empirical studies indicate that hydroponic systems can reduce water consumption by up to 90%, a critical factor in sustainable agriculture.
Additionally, the absence of soil minimizes the risk of soil-borne pathogens and pests, thereby reducing the need for pesticides.
Data suggest that plants grown hydroponically exhibit growth rates up to 50% faster than their soil-grown counterparts, attributed to the direct and efficient uptake of nutrients in a controlled environment.
Historical Context
Tracing its origins back to ancient civilizations, the practice of soilless cultivation has a rich historical context that underscores its evolution into modern hydroponic systems.
The Hanging Gardens of Babylon, dating back to 600 B.C., serve as an early example of hydroponic-like methods.
By the 17th century, Jan van Helmont‘s experiments demonstrated that plants absorbed nutrients from water, laying foundational knowledge.
In the 1930s, Dr. William F. Gericke coined the term ‘hydroponics‘ and advanced the technique by growing tomatoes in nutrient solutions.
Subsequent research, particularly during NASA’s Controlled Ecological Life Support System (CELSS) studies in the 1980s, further refined hydroponic technology.
These historical milestones collectively illustrate the progressive enhancement and application of hydroponics in plant biological research.
Basic Principles
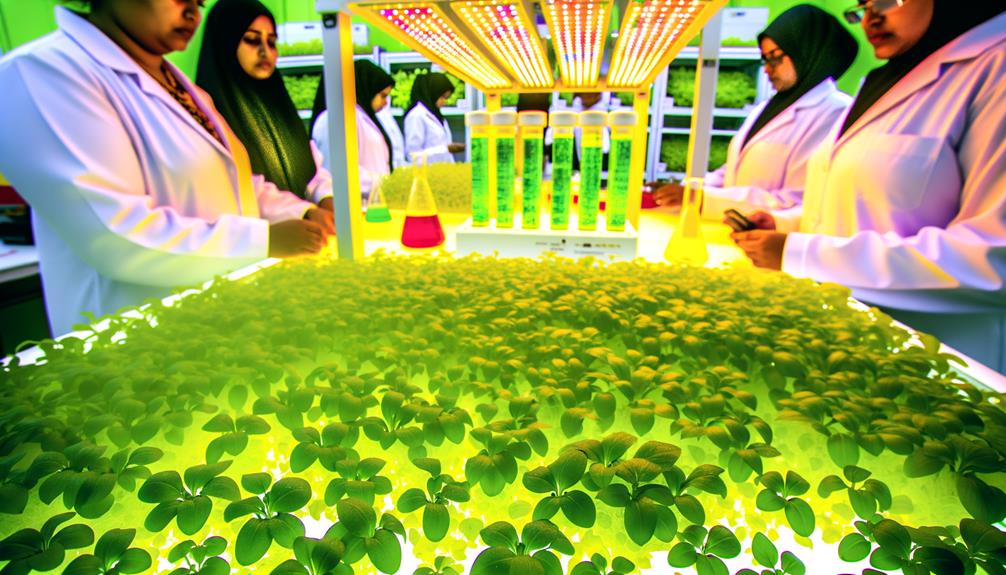
Fundamental to the understanding of hydroponic systems are the principles of nutrient solution management, root zone environment control, and the enhancement of light and temperature conditions. These principles are paramount for guaranteeing peak plant growth and development.
- Nutrient Solution Management: Precise formulation and regular monitoring of nutrient solutions are critical. Parameters such as pH and electrical conductivity (EC) must be meticulously managed to guarantee nutrient availability and uptake.
- Root Zone Environment Control: Adequate aeration and temperature regulation within the root zone are essential. Oxygenation prevents hypoxic conditions, while temperature control mitigates thermal stress.
- Enhancement of Light and Temperature Conditions: Correct light spectra and photoperiods, alongside temperature calibration, are indispensable for photosynthetic efficiency and metabolic processes.
These foundational principles underpin the efficacy and reliability of hydroponic systems in plant biological research.
Nutrient Management
Nutrient management in hydroponics is pivotal for optimizing plant growth and research outcomes, necessitating precise control over nutrient ratios and concentrations.
Monitoring nutrient levels through advanced sensors and analytic techniques guarantees the maintenance of ideal conditions.
Effective nutrient delivery systems, such as drip irrigation and nutrient film technique, are essential for consistent and uniform nutrient distribution.
Optimal Nutrient Ratios
Determining the ideal nutrient ratios in hydroponic systems is critical for maximizing plant growth and yield, requiring precise adjustments based on specific plant species and developmental stages.
Accurate nutrient management involves balancing macronutrients (N, P, K) and micronutrients (Fe, Mn, Zn, etc.) to guarantee optimal plant health.
Key considerations include:
- Species-Specific Requirements: Different plants have unique nutrient needs; for instance, leafy greens might require higher nitrogen levels, whereas fruiting plants need more potassium.
- Growth Stages: Nutrient ratios must be tailored to the plant’s growth phase—seedlings require different nutrient concentrations compared to mature plants.
- Nutrient Interactions: Understanding the synergistic and antagonistic interactions between nutrients is essential to prevent deficiencies or toxicities.
Implementing these parameters guarantees robust hydroponic crop production.
Monitoring Nutrient Levels
Effective nutrient monitoring in hydroponic systems relies on precise measurement techniques such as electrical conductivity (EC) and pH testing to guarantee ideal nutrient availability and uptake.
EC measurements, quantified in microsiemens per centimeter (μS/cm), provide data on the total ionic concentration of the nutrient solution, confirming that plants receive the correct balance of essential minerals.
Concurrently, pH levels, ideally ranging between 5.5 and 6.5, are monitored to optimize nutrient solubility and root absorption.
Advanced sensors and automated systems facilitate real-time data acquisition and adjustments, mitigating risks of nutrient imbalances.
Implementing these precision methodologies confirms robust plant growth, increased yield, and reproducible research outcomes, underlining the critical role of meticulous nutrient monitoring in hydroponic research.
Nutrient Delivery Systems
Advanced nutrient delivery systems play an essential role in hydroponic research by ensuring the precise and consistent supply of essential minerals to plants. These systems often employ sophisticated mechanisms to optimize nutrient uptake, thereby enhancing plant growth and development.
Key components include:
- Automated Dosing Units: These devices regulate the concentration of nutrients in the solution, ensuring real-time adjustments based on plant needs.
- Recirculating Systems: By recycling nutrient solutions, these systems maintain a stable nutrient profile while reducing waste.
- pH and EC Monitoring: Continuous pH and electrical conductivity (EC) sensors provide critical data for adjusting nutrient formulations, optimizing absorption efficiency.
Environmental Controls
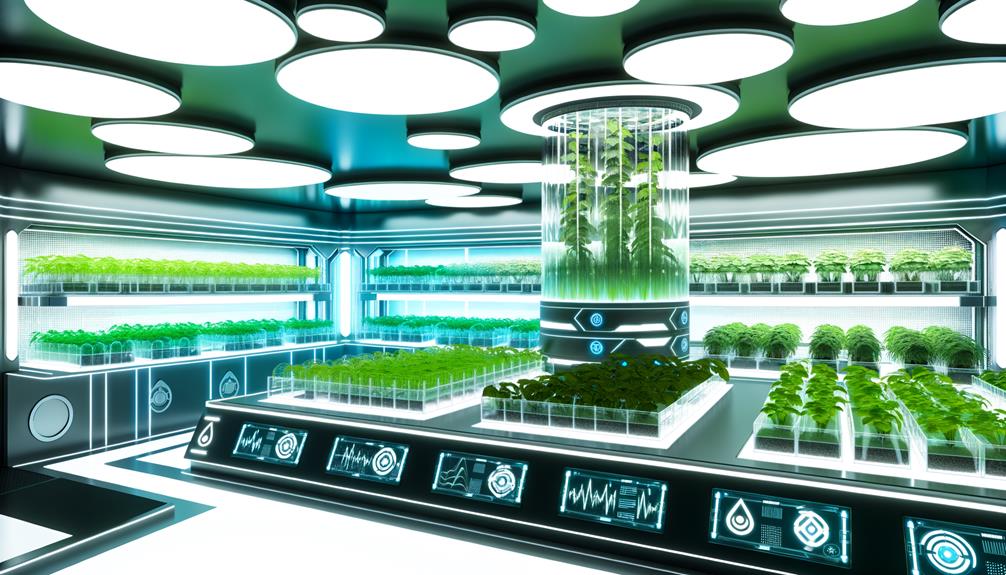
Environmental controls in hydroponics are critical for maintaining ideal growth conditions and maximizing plant health and yield. Key parameters such as temperature, humidity, CO2 concentration, and light intensity must be meticulously regulated.
Best temperature ranges between 20-25°C, while relative humidity should be maintained at 50-70% to prevent pathogen proliferation.
CO2 levels are typically enhanced to 800-1200 ppm to stimulate photosynthesis, thereby accelerating growth rates.
Light intensity, measured in micromoles per square meter per second (μmol/m²/s), must be tailored to the specific photosynthetic needs of the plant species, generally ranging from 400 to 700 μmol/m²/s.
Advanced environmental control systems employ sensors and automation to guarantee real-time adjustments, thereby achieving precise microclimatic conditions conducive to superior plant development.
Plant Physiology Insights
Understanding plant physiology within hydroponic systems is vital for maximizing nutrient uptake, growth rates, and overall plant health. This understanding is predicated on three significant insights:
- Nutrient Solution Composition: Precise control of macronutrients (N, P, K) and micronutrients (Fe, Mn, Zn) in the solution is essential for mitigating deficiencies and toxicities.
- Root Zone Environment: Parameters such as dissolved oxygen, pH, and electrical conductivity (EC) must be meticulously managed to guarantee ideal root function and nutrient absorption.
- Photosynthetic Efficiency: Light intensity, spectral quality, and photoperiod directly influence photosynthesis rates, thereby impacting biomass accumulation and yield.
Genetic Research
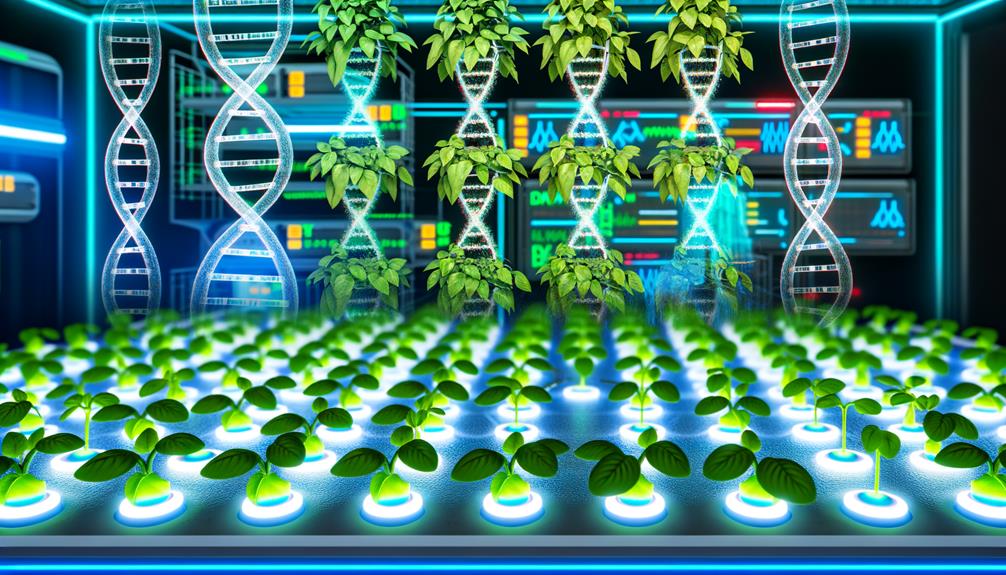
The utilization of hydroponic systems in genetic research offers precise control over environmental variables, thereby minimizing external influences on gene expression.
This method facilitates the accurate assessment of gene function and regulatory mechanisms under stress conditions.
Consequently, hydroponics serves as an ideal platform for elucidating plant stress responses and enhancing targeted genetic modifications.
Controlled Environment Benefits
Hydroponic systems enable precise control over environmental variables, offering a unique advantage for conducting genetic research on plant phenotypes and genotypes. This level of control facilitates the isolation of specific genetic traits by eliminating confounding factors related to soil variability and nutrient delivery inconsistencies.
The benefits of such controlled environments in genetic research include:
- Reproducibility: Consistent environmental conditions guarantee that experiments can be accurately replicated, enhancing the reliability of genetic studies.
- Parameter Manipulation: Researchers can meticulously adjust variables such as pH, nutrient concentration, and light exposure, providing valuable insights into gene-environment interactions.
- Stress Response Analysis: Controlled settings allow for the precise application of abiotic and biotic stressors, enabling detailed assessments of plant stress responses and adaptive genetic traits.
This precision fosters advancements in understanding plant genetics and breeding.
Enhanced Gene Expression
Often, hydroponic systems enable researchers to achieve more precise and enhanced gene expression profiling, providing critical data for understanding genetic regulatory mechanisms in plants.
By eliminating soil variability, hydroponics guarantees uniform nutrient delivery, reducing experimental noise. This controlled environment facilitates accurate quantification of transcriptional responses under varying conditions.
Utilizing technologies like RNA-seq, hydroponically grown plants exhibit distinct expression patterns, allowing for high-resolution mapping of gene networks.
Comparative studies reveal significant upregulation or downregulation of specific genes, elucidating pathways involved in growth, development, and metabolic processes.
Hydroponic systems also support real-time monitoring of gene expression dynamics, offering insights into temporal regulatory mechanisms.
Consequently, this method proves indispensable for advancing genetic research and functional genomics in plant biology.
Stress Response Studies
Leveraging the controlled conditions of hydroponic systems, researchers can meticulously investigate plant stress responses at the genetic level, ensuring accurate assessment of transcriptional changes under abiotic and biotic stressors. This precision is essential for elucidating the intricate molecular pathways involved in stress tolerance.
Key aspects include:
- Gene Expression Profiling: Utilizing RNA sequencing (RNA-Seq) to identify differential gene expression patterns in response to specific stressors.
- Mutant Analysis: Employing CRISPR-Cas9 and other gene-editing tools to generate mutants, allowing the functional dissection of stress-related genes.
- Stress Marker Quantification: Measuring physiological markers like reactive oxygen species (ROS) and proline to correlate with transcriptional data.
These methodologies enable a thorough understanding of the genetic mechanisms underpinning stress resilience, facilitating the development of robust plant varieties.
Biochemical Studies
Biochemical studies leveraging hydroponic systems allow for precise control over nutrient delivery, enabling detailed analysis of plant metabolic pathways.
The absence of soil mitigates external variable interference, ensuring reproducibility and accuracy in experimental conditions.
For instance, the quantification of secondary metabolites like flavonoids and alkaloids can be meticulously monitored through HPLC and GC-MS techniques.
In addition, hydroponics facilitates isotopic labeling experiments, which elucidate nutrient uptake and assimilation kinetics.
Enzyme activity assays, such as nitrate reductase and glutamine synthetase, benefit from the homogenous nutrient environment, providing consistent data.
Moreover, hydroponic systems enable controlled stress induction, such as salinity or metal toxicity, allowing for precise biochemical response profiling.
This high degree of control is indispensable for advancing our understanding of plant biochemistry.
Reproducibility in Experiments
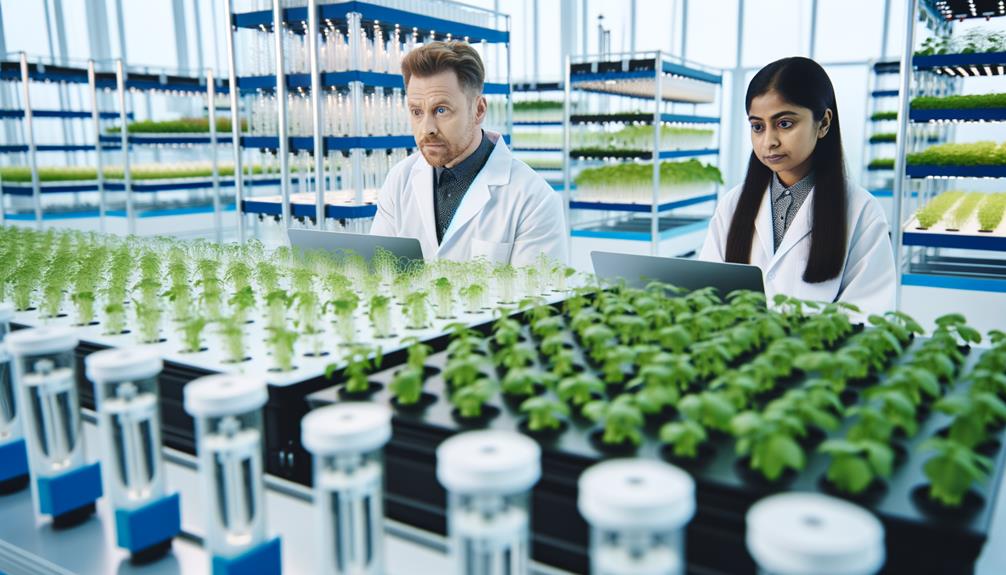
Ensuring reproducibility in hydroponic plant research necessitates the standardization of growth conditions to foster consistent experimental outcomes.
By minimizing environmental variability, researchers can reduce confounding factors that compromise data integrity.
Consequently, achieving high data consistency and accuracy becomes feasible, facilitating reliable comparisons across different studies.
Standardizing Growth Conditions
Standardizing growth conditions is essential for achieving reproducibility in hydroponic plant research experiments.
Precise control of environmental parameters guarantees consistent data and minimizes experimental variability. Key factors include:
- Nutrient Solution Composition: Maintaining a consistent nutrient profile is necessary. Deviations in macronutrient and micronutrient concentrations can greatly impact plant growth and physiological responses.
- pH Levels: pH stability is critical for nutrient uptake. A pH range of 5.5-6.5 is generally ideal, but must be rigorously monitored and adjusted to prevent nutrient lockout or toxicity.
- Light Intensity and Photoperiod: Uniform light conditions, including intensity (measured in µmol/m²/s) and duration, are essential for guaranteeing comparable photosynthetic activity and growth rates across experiments.
Minimizing Environmental Variability
To achieve reproducibility in hydroponic plant research, it is imperative to rigorously control and monitor all environmental factors that could introduce variability into the experimental outcomes. Key variables include light intensity, temperature, humidity, pH levels, and nutrient concentrations. Precise calibration and continuous monitoring of these parameters are essential to guarantee uniform growth conditions across different experimental setups. Utilizing automated systems for environmental control can greatly reduce human error and enhance reproducibility.
Environmental Factor | Control Method |
---|---|
Light Intensity | LED grow lights, timers |
Temperature | HVAC systems, sensors |
Humidity | Dehumidifiers, sensors |
Nutrient Concentration | Automated dosing systems |
Adhering to stringent control protocols mitigates external influences, thereby yielding consistent and reliable data.
Data Consistency and Accuracy
Achieving data consistency and accuracy in hydroponic plant research necessitates rigorous standardization of experimental protocols and meticulous documentation of all procedural variables.
Precision in data collection is paramount to guarantee reproducibility and reliability of results. The following key factors must be adhered to:
- Nutrient Solution Composition: Strictly control the concentration and composition of nutrient solutions to avoid variability in plant growth conditions.
- Environmental Control: Maintain consistent environmental parameters (temperature, humidity, light intensity) using automated systems to minimize extraneous influences.
- Documentation and Calibration: Employ detailed record-keeping and routinely calibrate measurement instruments to maintain data integrity.
Adhering to these practices guarantees that experimental outcomes are both replicable and scientifically valid, facilitating advancements in plant biological research.
Future Prospects
Advancements in hydroponic technology hold the potential to revolutionize plant research by enabling precise control of environmental variables and nutrient delivery systems. Emerging innovations such as automated nutrient dosing, real-time pH and EC monitoring, and advanced LED lighting systems offer unparalleled data accuracy and reproducibility.
These technologies facilitate the study of plant physiology under standardized conditions, eliminating soil-related variables. Additionally, integration with AI and machine learning algorithms could optimize growth parameters and yield predictive models for plant responses.
Future research may leverage hydroponics to explore genetic expressions and stress responses, enhancing our understanding of plant biology. This methodological precision could accelerate breakthroughs in crop improvement, sustainable agriculture, and climate resilience, marking a pivotal shift in plant biological research.
Conclusion
Hydroponics presents a robust methodology for plant biological research, offering precise control over environmental variables and nutrient delivery. This controlled setting allows researchers to manipulate factors such as light, temperature, and nutrient composition to study plant responses with high accuracy. Scientists can explore hydroponic plants’ immunity by examining how different nutrient solutions affect disease resistance and overall plant health. Additionally, hydroponics minimizes soil-borne pathogens, providing a cleaner environment to investigate plant-microbe interactions.
This system facilitates reproducibility and enhances the accuracy of genetic and biochemical studies.
For instance, a study conducted at the University of Arizona demonstrated a 30% increase in the growth rate of lettuce using hydroponic systems compared to traditional soil cultivation, underscoring the potential for significant advancements in agricultural and biological research through hydroponics.
Future prospects include expanding genetic research and optimizing nutrient formulations.